LEONID DAILY NEWS: November 20, 2000
A rather imaginative but very controversial
identification of the 3.4 micron feature in Leonid persistent
trains, discovered by Russell et al. (see Nov. 13 news item),
was made by Chandra Wickramasinghe and Fred Hoyle of the Cardif Centre for Astrobiology
in a paper posted on the internet. The paper is reproduced below.
A BACTERIAL "FINGERPRINT" IN A LEONID METEOR TRAIN
Chandra Wickramasinghe and Fred Hoyle
Cardiff Centre for Astrobiology
School of Mathematics, Senghennydd Road
PO Box 926, Cardiff CF2 4YH, UK
E-mail: wickramasinghe [at] cf.ac.uk
Abstract
A recently observed broad 3.4m
m spectral "fingerprint" in a persistent Leonid meteor train at a height of 83km is likely to be due to emission of surrounding mesospheric bacteria heated by the passage of an incandescent fireball.
______________
Russell et al (2000) have recently published infrared spectra of persistent meteor trains that were observed during the Leonid meteor shower of November 1999. The train left by a minus13 magnitude Leonid fireball at a height of 83.2±
1 km, yielded a spectrum that contained an enigmatic broad CH emission feature superposed on an underlying continuum. There were also other features attributed to CO2 and CO, but no 10m
m silicate feature was seen.
The 3.4m
m feature (enlarged from a Figure 2b in Russell et al) is reproduced as the solid curve in Fig.1. The authors discuss the possibility of organic molecules forming in recombination reactions within the meteor train, but in view it is more reasonable to suppose that the infrared emission arose from organic particles already present in the surrounding mesosphere that were heated by the meteor train. The uncanny resemblance of the solid curve in Fig. 1 to the 3-3.5m
m spectrum of Comet Halley obtained on 31 March 1986 (D.T. Wickramasinghe et al, 1986) prompted us to explore this comparison in a little more detail.
If k
(l
) is the mass absorption coefficient of the emitting dust at wavelength l
and T is the temperature of a dust grain heated in the meteor train, the resulting flux at the Earth is
Fl
= constant. k
(l
) Bl
(T) .............(1)
where Bl
(T) is the Planck function. The function k
(l
) is proportional to the optical depth t
(l
) measured for a laboratory sample of bacteria, thus giving
Fl
= constant. t
(l
) Bl
(T) ............(2)
for the observable flux from heated grains. For the optical depth t
(l
) we take Al-Mufti's (1985) measurements for E.coli that had been dessicated by heating to 350K (See also Wickramasinghe et al, 1986). Table 1 sets out the values of relative flux generated by equation (2) with a temperature T set equal to 400K.
Table 1
3.2-3.5m
m emission from bacterial grains heated to 400K
l
(m
m) |
t
(l
) |
Fl
|
3.282
3.310
3.324
3.338
3.352
3.366
3.380
3.394
3.408
3.429
3.436
3.450
3.464
3.478
3.492 |
0.172
0.161
0.161
0.163
0.184
0.226
0.240
0.252
0.276
0.240
0.215
0.185
0.161
0.158
0.157 |
0.503
0.495
0.508
0.525
0.606
0.765
0.829
0.891
1.000
0.899
0.814
0.718
0.640
0.641
0.648 |
The values of Fl
in Table 1 (suitably scaled) are plotted as the dashed curve of Fig.1. The agreement between the meteor-wake infrared emission and the bacterial model is seen to be satisfactory. It is clear from the width of the observed band that small organic molecules in the gas phase cannot provide a viable explanation for the band. The optical properties of a mixture of organic polymers suitably assembled so as to mimic the bacterial spectrum appears to be called for, and surely the simplest way to achieve this is by taking the bacterial model itself. The small degree of mismatch between the data and the model seen in Fig.1 is unlikely to be significant. It could be due
partly to the simplicity of our modelling procedure, and partly also to the inadequate quality of the data. An improved fit to the data as it stands is, however, possible if we consider a distribution of grain temperatures, which is more realistic than the single temperature used in our calculation.
The conclusion to be drawn from Fig. 1 is that the meteor trail "fingerprint" at 3.4m
m is most likely due to a population of bacterial particles in the mesosphere that have been transiently heated to temperatures T ~ 400K.
The minus 13 visual magnitude stated for the meteor implies a considerable mass of incandescent material, possibly upwards of 105 g, is involved (Millman and McKinley, 1963). If such a large mass traversed the atmosphere as a single coherent chunk the resulting fireball/meteor train should have been seen at a considerably lower altitude than 80km. The observations of Russell et al (2000) imply a loose fluffy meteoroid aggregate that became separated into millimetre sized pieces at great heights, which then burnt out at ~ 80km. The total kinetic energy associated with say 105 g of incoming material would be initially distributed in a volume of mesospheric air at h=83km of ~ 105/r
»
105 /10-8 cm 3 = 1010 litres. Part of this energy would become available to heat bacterial particles in the mesosphere in an even larger volume around the meteor train. It would be difficult to argue that bacteria from the surface of the Earth would be lofted to heights of some 83km into the mesosphere, at any rate in adequate quantity to explain the observations. On the other hand, a population of mesospheric bacteria could form part of the known daily influx of cometary organic material that reaches the Earth. The new data can then be seen as further evidence in support of panspermia (Hoyle and Wickramasinghe, 2000).
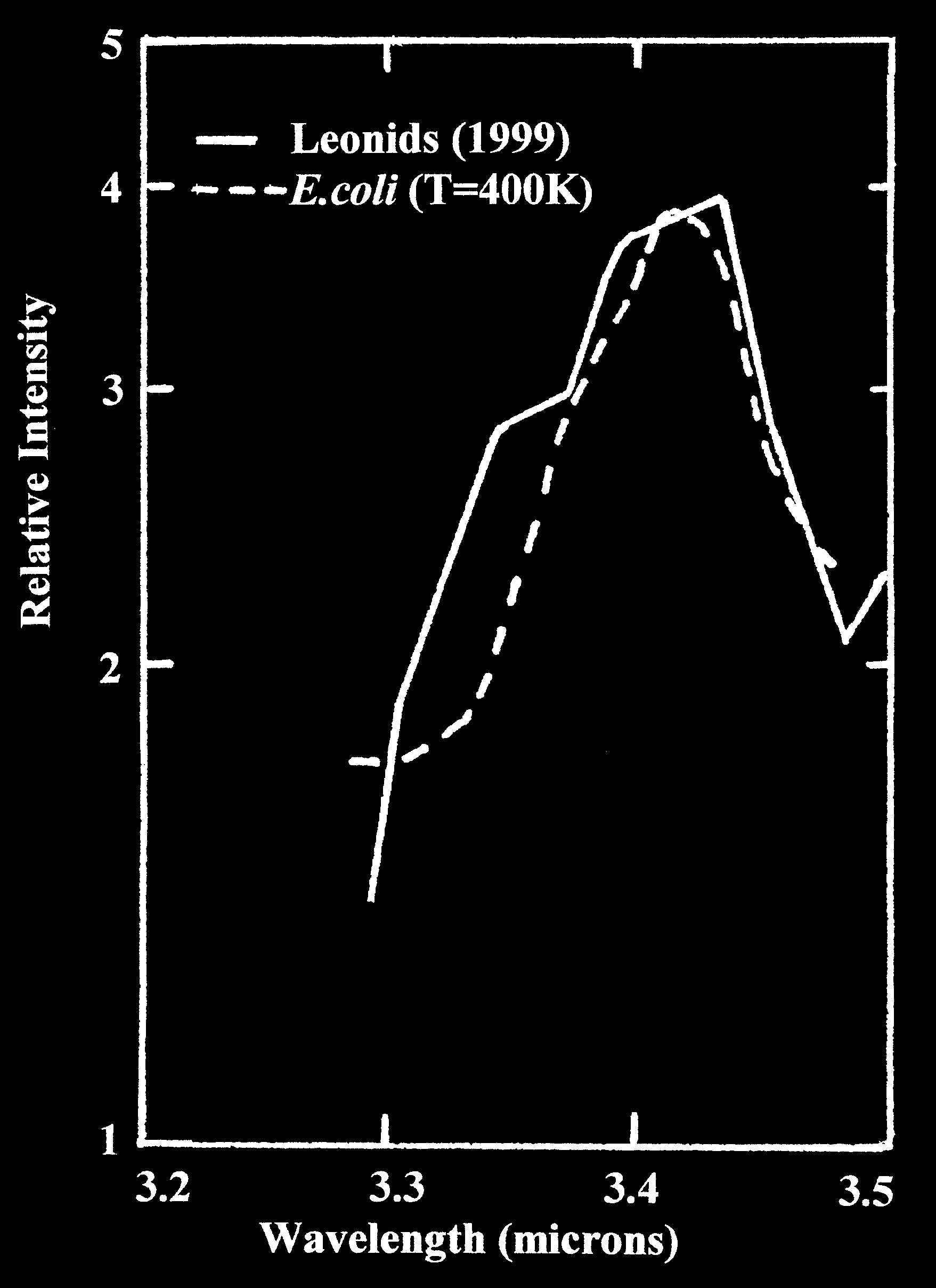
Figure 1. The infrared emission over the 3.2-3.5m
m waveband observed by Russell et al (2000) for a Leonid meteor train (solid curve) compared with the flux calculated for a bacterial grain heated to 400K (dashed curve).
References
Al-Mufti, S.: 1985. PhD Thesis, University College Cardiff
Hoyle, F. and Wickramasinghe, N.C.: Astronomical Origins of Life: Steps towards panspermia (Kluwer Academic Publishers, Dordrecht)
Millman, P.M. and McKinley, D.W.R.: 1963. In The Moon, Meteorites and Comets (eds. B.M. Middlehurst and G.P. Kuiper) (Univesity of Chicago Press)
Russell, R.W., et. al, 2000. Earth, Moon and Planets,
Wickramasinghe, D.T., Hoyle, F., Wickramasinghe, N.C. and Al-Mufti, S.:1986. Earth, Moon and Planets, 36, 295-299.
Previous news items:
Nov. 20 - A bacterial fingerprint?
Nov. 15 - HCN disappears mysteriously
Nov. 14 - Meteor shower from space
Nov. 13 - Organic fingerprint
Nov. 12 - Train airglow chemistry
Nov. 11 - Hard bits and persisting glows
Nov. 10 - Meteoroid debris detected
Nov. 09 - New meteor picture
Nov. 08 - Spin city
Nov. 07 - Meteors affect atmospheric chemistry
Nov. 06 - Listen to this!
Nov. 04 - Fear of heights?
Nov. 03 - The pale (infra-red) dot
Nov. 02 - Twin showers
Nov. 01 - Leonids approaching Earth
Oct. 31 - Prospects for Moon Impact Studies
Oct. 30 - Comet dust crumbled less fine
Today's news
Results of Leonid storm research
have appeared in a special issue of the peer-reviewed journal
"Earth, Moon and Planets", published by Kluwer Academic Publishers, the Netherlands.
|
|